Quote of the Day
In order to write about life, first you must live it.
— Ernest Hemingway
Introduction

Figure 1: Stromatolites in Shark Bay, Australia (Source:Wikipedia).
As my regular readers can tell, I do not passively sit and watch television. While I am watching a program (history or science-oriented, nothing else), I have my computer right there and I actively research what is being said during the program. Last weekend, I was watching an interesting program on the History channel called "How the Earth was Made". This particular program was about the formation of the Earth and it contained an excellent section on the generation of atmospheric oxygen (transcript of program). In my opinion, the star of the show was a little rocky structure called a stromatolite (Figure 1). A stromatolite is a layered, rock-like structure formed when shallow-water sediments are trapped in films of microorganisms.
Stromatolites can get quite large, such as this two-ton one reported here.
What piqued my mathematical interest was the following statement.
Over a period of 2 billion years, countless generations of stromatolites pumped out over 20 million billion tons of oxygen.
I was wondering if I could gain some additional insight into this number. In the main body of this post, I will look at how much oxygen is in our atmosphere today. In the appendix, I will examine how to estimate the total amount of oxygen over time that has been released on Earth. These will be rough order of magnitude calculations. Let's dig in ...
Analysis
Earth's Atmosphere and Oxygen
Initially, the Earth's atmosphere had very little oxygen (~25 million times less than today) – then came the "Great Oxidation Event", which started some 2.3 billion years ago. During this time, atmospheric oxygen levels rose dramatically. The oxygen was being pumped out by organisms like stromatolites. We know that this oxygen ended up in places other than the atmosphere. For example, I live in Minnesota where iron oxide is all over the northern half of the state – the amounts are staggering. There are also large amounts of oxygen dissolved in the ocean and embedded in the crust. This article discusses three mechanisms for incorporating atmospheric oxygen in the crust.
- iron reacting with oxygen-rich seawater and precipitating out.
- oxygen-rich water in seafloor sediments drawn into the crust.
- oxygen-rich sulfates in undersea hot springs reacted with iron in seafloor sediments.
How Much Oxygen is in the Atmosphere Today?
We live at the bottom of a sea of air. When we measure air pressure, we are really measuring the weight of the mass of air above that point. We can roughly calculate the mass of atmosphere by multiplying the air pressure times the area that pressure covers. We will break the Earth up into two parts: land and ocean.
We need to collect a few facts before we start our analysis.
- 20.95% oxygen by volume (Source - dry air value, I am ignoring water vapor)
- 70.8% of the Earth's surface is covered by water, 29.2% by land (Source)
- The mean radius of the Earth is 6,371.0 km (Source)
- The mean air pressure at sea level is 101.325 kP or 14.7 psi (Source)
- The mean elevation of the continents is 840 meters (Source).
- The mean air pressure at 840 meters is 91.633 kP or 13.3 psi (Source - equation used in Fig. 2)
With these facts in mind, it is now time to pull out the mathematical artillery (i.e. Mathcad).
Percentage of Oxygen By Mass
For ideal gases, the volume fraction and mole fractions are equal. The real atmosphere is not ideal, so let's go and compute the percentage of oxygen in the atmosphere by mass. To perform this calculation, we can use the gas composition of the atmosphere by volume and the molecular weights of these gases to compute the percentage of oxygen by mass. Equation 1 illustrates the calculation.
Eq. 1 |
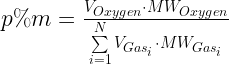 |
I used a table from the Wikipedia and did the Equation 1 calculation as illustrated in Figure 2.

Figure 2: Calculating the Percentage of Oxygen in the Atmosphere by Mass.
Mass of Oxygen in the Atmosphere
Figure 3 shows the calculation for the total mass of oxygen in today's atmosphere.

Figure 3: Calculating the Mass of Oxygen in the Atmosphere.
Conclusion
Let's see if I can check my answers. According to the National Center for Atmospheric Research, the total mass of the Earth's atmosphere is 5.1353·1015 metric tons. So I have good agreement there. My estimate for the mass fraction of oxygen in the Earth's atmosphere (23.1%) equals that stated in the Wikipedia, so I am good there. My estimate for the total mass of O2 in the atmosphere is 1.2 million billion metric tons, which agrees with this reference. So overall the analysis is pretty close.
The stromatolites alone produced 20 million billion tons (possibly more, see Appendix). The majority of the oxygen produced by stromatolites has apparently gone somewhere other than the atmosphere. In fact, there are enormous amounts of atmospheric oxygen that ended up chemically tied up in rocks. Consider the following quote (Source).
While photosynthetic life reduced the carbon dioxide content of the atmosphere, it also started to produce oxygen. For a long time, the oxygen produced did not build up in the atmosphere, since it was taken up by rocks, as recorded in Banded Iron Formations (BIFs) and continental red beds. To this day, the majority of oxygen produced over time is locked up in the ancient "banded rock" and "red bed" formations. It was not until probably only 1 billion years ago that the reservoirs of oxidizable rock became saturated and the free oxygen stayed in the air.
Figure 4 is from the Wikipedia and does a nice job illustrating the Earth's O2 level over time. The red and green lines illustrate the range of estimates for the percentage of O2.

Figure 4: Earth's Atmospheric Oxygen Percentage Versus Time.
There are some folks that claim the high oxygen levels in the past may have played a role in making dinosaurs so large.
Appendix A: Interesting Quote on Stromatolite-Produced Oxygen
Figure 5 shows where the photosynthesized oxygen went (Source). Note that this reference assumes that the total amount of photosynthesized mass of O2 on Earth is 3x1022 grams (=30 million billion metric tons). Clearly, estimates for the amount of photosynthesized O2 vary a bit.

Figure 5: Cumulative History of O2 by Photosynthesis Through Geologic Time.
The following quote (source) describes how most of the oxygen was chemically bound to the Earth and the rest was released to the air.
A sort of mass balance for atmospheric oxygen (including that dissolved in the sea) is shown in Figure 13.8 [my Figure 5]. The excess oxygen depends on a corresponding accumulation of organic matter (plus biologically reduced sulphur and methane) that has become buried in sedimentary deposits. About 58% and 38% of this oxygen has been used for the oxidation of iron and sulphur, respectively during the 3.5 - 3.8 billion years of organic photosynthesis. The remaining 4% (about 1.2x1015 tonnes) is found as free O2. The turnover rate of this atmospheric oxygen is only about 4000 years and is caused by organic photosynthesis and respiration processes that almost balance so that the biological turnover is vastly faster than the slow accumulation of organic matter. Given the crude estimates in Figure 13.5. there is altogether an excess of 3x1016 tonnes of oxygen (of which the greater part is in the form of oxidized iron and sulphur and only 4% as O2). Over roughly four billion years this corresponds to a net accumulation of 7.7x106 tonnes per year. The annual turnover due to photosynthesis and respiration corresponds to (1.2x1015[tonnes])/4000 [years] = 3x1010[11] tonnes O2 per year; that is production and respiration is about 20,000[36,500] times faster than the slow accumulation of organic matter in sedimentary rocks. This calculation, of course, assumes that both the production rate and the rate of accumulation of un-mineralized organic matter have been constant over geological time. Some evidence indicates that this has been the case since roughly the second half of the Precambrian.
As you can see from the quote, the calculation of total O2 production over the history of the Earth requires a number of assumptions.
I have noticed some minor math errors (see highlights) in the quote given above – minor in the sense that they do not change the result qualitatively, but I do see some calculation issues. Figure 6 shows my version of the calculations using the data given above. In the calculation, I assume that the period of organic photosynthesis was 3.65 billion years (the mean of the range given in the quote).

Figure 6: Detailed Calculation Using Given Data.
Save